RESEARCH
December 2019
Stanisa Raspopovic and Francesco Maria Petrini
Challenges of leg amputees in everyday life
Leg amputees, while walking, do not trust the prosthesis and rely too much on the healthy leg, reducing mobility, and risking falls [1]. The prosthesis, not being connected with the brain, doesn’t feel as a part of their body.
Lower limb amputees, wear commercial prostheses that do not give any information about the interaction of the device with the ground or its movement. Because of the lack of sensory feedback, amputees risk falls, with consequent loss of confidence in the use of the prosthesis and excessive relying on the intact leg [2]. Such asymmetrical walking increases fatigue [3] and reduces mobility [2]. The lack of sensory information from the prosthesis forces the person to continuously monitor the device, increasing the brain effort during walking [4], and resulting in the important cognitive load. Finally, since the physiological link between the phantom foot and brain is missing, their brain develops the maladaptive plasticity resulting in amputees feeling pain from the missing leg called phantom limb pain-PLP [5].
These factors create costs to healthcare systems, which have to treat falls, pain, and complications connected to low mobility, and reduce sales of prosthesis manufacturers. Only in Europe and USA, approximately 4 million people have this condition.
The first “feeling” leg
Recently the more invasive, implantable devices, aiming to the intimate contact with residual nerves, have entered into the scene of neurorehabilitation [9-10]. We have developed a lower limb neuroprosthesis that allowed 3 amputees to feel sensations of touch and movement from the missing extremity as if they were coming from the device itself [11-12].
The neuroprosthesis (Fig 1) is constituted by a commercial lower limb prosthesis equipped with sensors under the foot sole and in the knee, a microcomputer and a stimulating system. The sensors readouts are acquired and recorded by the microcomputer which transduces them in stimulation instructions for the stimulator, which is placed out of the body. The signals from the insole and prosthetic knee sensors are translated in impulses of current, the language of the human nervous system, which are delivered to the residual peripheral nerve through tiny electrodes [10], implanted transversally into the nerve itself. The stimulator delivers electrical current trains to implantable nerve electrodes to which it connects by small cables passing through the skin. Then, nature does the rest: the signals from the residual nerves are conveyed to the brain of the person, which is able to perceive what happens at the prosthesis and to adjust the walking accordingly. The machine and the body are finally re-connected.
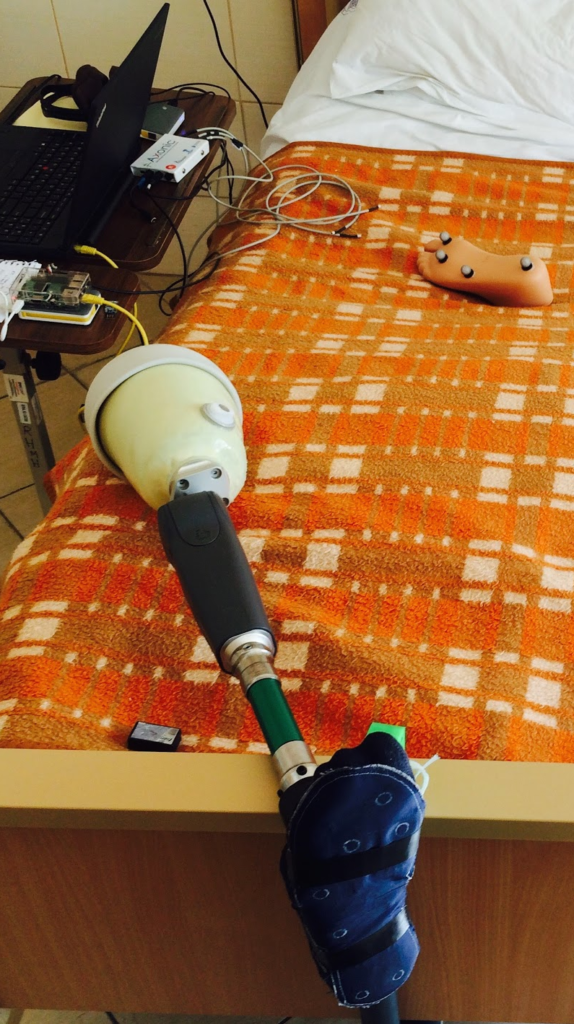
Fig.1. The neuroprosthesis [11] is constitutited of the sensorized insole, which transmits wirelessly the information to the system controller, that triggers the neural stimulation.
Transforming artificial signals into natural ones
In order to calibrate the neuroprosthesis – i.e. determine the electrode channels to connect to the prosthesis sensors and the stimulation parameters – the response of the patients to the current stimulation is assessed during the first month after implant. Trains of current are delivered through all the 64 implanted electrode channels into the nerve, while stimulation parameters (intensity, duration and frequency) are varied.
Sensations of touch, pressure, vibration (as well as paresthesia or electricity) were elicited from more than 20 positions of the phantom foot sole, and of contraction or solicitation from the muscles of the missing leg.
The first time the subjects used the neuroprosthesis they felt as it was very similar to their missing leg (Supplementary video 1 from [11]). Indeed, no training was needed to understand that sensations of touch, vibration or pressure were elicited by the interaction of the prosthetic foot sole with the ground and that sensations of muscle contraction were coming from the movement of the prosthetic knee.
When the volunteers were asked to recognize blindfold the touch under the prosthetic foot (Fig.2) or the flexion/extension of the prosthetic knee or the two conditions simultaneously they achieved an average of more than 80% successful responses.
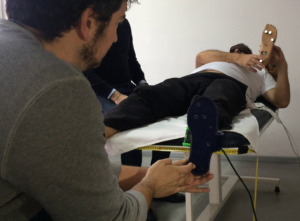
Fig.2. The touch recognition task [12]: user is recognizing and replicating the position on which he has been touched on the artificial foot.
They climbed/descended stairs around 30 % quicker with sensations restored, than without them. When they stepped outside of the lab, they moved over uneven and very hard to walk sandy terrains faster with the artificial sensations than without them. The neuroprosthesis sensations doubled confidence in the use of the prosthesis and enabled the subjects to feel the prosthesis as if it was part of their body.
What about the brain?
The prosthesis, not being connected with the brain, doesn’t feel as a part of their body. In order to test the hypothesis that the nerve integration of the prosthesis would boost also the brain perception, we ideated 2 quantitative tests.
In first we assessed the level of embodiment [12] after the tests performed with and without feedback enabled. Questionnaires and the measurement of the proprioceptive drift robustly shown that they felt the prosthesis as more being their body with the feedback enabled.
During the second set of tests the dual task [11-12] was implemented, during which they had to walk while listening the tones, and paying attention on higher ones. During this task the ERP component of EEG was measured in order to unveil if mental budget available for the walking was higher or lower with/without sensory feedback. It resulted that with the feedback users were having lot of mental budget available, as when counting the tones while sitting, while in the case of no-feedback they had small available mental resources. Therefore, the amputees can walk freely while thinking about different activities other than controlling the device.
Brain activity measurements and psychophysical tests revealed that the neuroprosthesis is perceived as an extension of the body, as a real limb. The connection between the machine and nerve is fundamental to achieve this.
“You don’t need to concentrate to walk, you can look forward and step, you don’t need to look where your leg is to not fall,” said one of the volunteers in the study.
The bionic leg integrated with the residual nerve of amputees, enables the brain to accept it as the continuation of the natural leg, and this is essential for higher confidence of the users, and a future wide-spread of these technologies.
Clinically important PLP and metabolic cost diminishments
Walking with neurofeedback resulted to be physically much less demanding, as shown by the significant reduction in the volunteers’ oxygen consumption while walking. This is essential result since the amputees have more than a 100% higher risk of getting a heart attack. With continuous use of this system we assume that this risk will be diminished.
The interface with the nervous system can also be used to stimulate the nerves independently of the prosthesis. Before they started the trial, the volunteers complained of phantom limb pain. Over the course of a one-month therapy programme with neurostimulation, the pain was significantly reduced. This holds potential to diminish the use of opioids and better life quality of amputees.
Translation to all users
The results of this clinical trial represent the first drive to bring the sensory feedback restoration through direct nerve stimulation to clinical adoption. Lower limb amputees would have a solution to phantom pain and the limitations to the use of their currently available leg prostheses.
Prostheses manufacturers would have a reply to their continuous search of innovative technologies to upgrade their products. Healthcare systems would reduce the costs of treating falls, phantom pain and complications of a sedentary lifestyle.
A longer investigation with in-home assessments and a greater number of volunteers is needed, in order to provide more robust data that can be used to draw more significant conclusions. For the time-limited clinical study, the signals from the prosthesis were sent along cables through the skin to the electrodes in the thigh. This meant that the volunteers had to undergo regular medical examinations. To eliminate this need, a fully implantable system needs to be developed.
References:
- C. Miller, M. Speechley, B. Deathe, The prevalence and risk factors of falling and fear of falling among lower extremity amputees, Arch Phys Med Rehabil 82, 1031–1037 (2001).
- Nolan, A. Wit, K. Dudziñski, A. Lees, M. Lake, M. Wychowañski, Adjustments in gait symmetry with walking speed in trans-femoral and trans-tibial amputees, Gait Posture 17, 142–151 (2003).
- L. Waters, J. Perry, D. Antonelli, H. Hislop, Energy cost of walking of amputees: the influence of level of amputation, J Bone Joint Surg Am 58, 42–46 (1976).
- M. Williams, A. P. Turner, M. Orendurff, A. D. Segal, G. K. Klute, J. Pecoraro, J. Czerniecki, Does having a computerized prosthetic knee influence cognitive performance during amputee walking?, Arch Phys Med Rehabil 20 87, 989–994 (2006).
- Flor, H., Nikolajsen, L. & Staehelin Jensen, T. Phantom limb pain: a case of maladaptive CNS plasticity? Nature Reviews Neuroscience 7, 873–881 (2006).
- Rusaw, K. Hagberg, L. Nolan, N. Ramstrand, Can vibratory feedback be used to improve postural stability in persons with transtibial limb loss?, Journal of rehabilitation research and development 49, 1239–1254 (2012).
- Dietrich, S. Nehrdich, S. Seifert, K. R. Blume, W. H. R. Miltner, G. O. Hofmann, T. Weiss, Leg Prosthesis With Somatosensory Feedback Reduces Phantom Limb Pain and Increases Functionality, Front Neurol 9, 270 5 (2018).
- Crea, B. B. Edin, K. Knaepen, R. Meeusen, N. Vitiello, Time-Discrete Vibrotactile Feedback Contributes to Improved Gait Symmetry in Patients With Lower Limb Amputations: Case Series, Phys Ther 97, 198–207 (2017).
- Raspopovic, F. M. Petrini, M. Zelechowski, G. Valle, Framework for the development of neuroprostheses: from basic understanding by sciatic and median nerves models to bionic legs and hands, Proceedings of the IEEE 105, 34–49 (2017).
- M. Petrini, G. Valle, I. Strauss, G. Granata, R. D. Iorio, E. D’Anna, P. Čvančara, M. Mueller, J. Carpaneto, F. Clemente, M. Controzzi, L. Bisoni, C. Carboni, M. Barbaro, F. Iodice, D. Andreu, A. Hiairrassary, J. L. Divoux, C. 35 Cipriani, D. Guiraud, L. Raffo, E. Fernandez, T. Stieglitz, S. Raspopovic, P. M. Rossini, S. Micera, Six-months assessment of a hand prosthesis with intraneural tactile feedback, Annals of Neurology (2018)
- Petrini FM, Bumbasirevic M, Valle G, Ilic V, Mijović P, Čvančara P, Barberi F, Katic N, Bortolotti D, Andreu D, Lechler K, Lesic A, Mazic S, Mijović B, Guiraud D, Stieglitz T, Alexandersson Á, Micera S, Raspopovic S: Sensory feedback restoration in leg amputees improves walking speed, metabolic cost and phantom pain. Nature Medicine 2019, doi: 1038/s41591-019-0567-3 [http://dx.doi.org/10.1038/s41591-019-0567-3]
- M. Petrini, G. Valle, M. Bumbasirevic, F. Barberi, D. Bortolotti, P. Cvancara, A. Hiairrassary, P. Mijovic, A. Ö. Sverrisson, A. Pedrocchi, J.-L. Divoux, I. Popovic, K. Lechler, B. Mijovic, D. Guiraud, T. Stieglitz, A. Alexandersson, S. Micera, A. Lesic, S. Raspopovic, Enhancing functional abilities and cognitive integration of the lower limb prosthesis. Sci. Transl. Med. 11, eaav8939 (2019)
Dr. Stanisa Raspopovic obtained his MS in biomedical engineering at the University of Pisa, Italy. He then carried out research work at the Scuola Superiore Sant’Anna Biorobotics Institute, and received his PhD in 2011. During the PostDoc at EPFL Lausanne, he was guiding the pioneering activities in the sensory feedback restoration in upper limb amputees. Recently he joined ETH Zurich, where he runs the lab, working on the sensorimotor rehabilitation of the leg amputees and diabetics, with particular emphasis on the peripheral neural encoding and brain mechanisms involved.
Dr. Francesco Maria Petrini graduated cum laude in biomedical engineering in 2010 at the University of Tor Vergata, Rome. He received a PhD in biomedical engineering from Campus Bio-Medico University of Rome in 2015, with a thesis on neuroprosthetic applications for amputees. He has been visiting student and then postdoc at the Swiss Federal Institutes of Technology of Lausanne between 2013 and 2019. He is senior scientist at the Swiss Federal Institute of Technology in Zurich since 2018. His interests are in the development of novel interfaces to stimulate the peripheral nervous system to restore lost abilities following nerve damages, amputations or pathologies.